|
Overview:
The development of technologies that do not rely on fossil fuels is a major challenge facing society today. Our research program is aimed at the development and validation of models for the combustion properties of alternative and sustainable (bio-derived) fuels through a complementary experimental, computational, and analytical modeling approach. We are also investigating flames of a mixture of solid and gaseous fuels, combustion in heat recirculating burners for Stirling engine generators, and the reaction of metal-water mixtures for hydrogen production.
Check out some of the research vidoes on YouTube
Graduate Research Projects:
Premixed flame studies of alternative and traditional fuels:
We study the combustion of fuel-oxidizer mixtures over a range of equivalence ratios and flow conditions for a variety of fuels, from petroleum-derived jet fuel to biofuels. Using an impinging-jet experimental apparatus, we are able to generate a flat, stationary flame, ideal for experimental study. The flowfield through the flame is measured using laser visualization techniques (particle image velocimetry). Using spectroscopy, chromatography and laser-induced fluorescence, the consumption of reactants and the profiles of species and the temperature through the experimental domain can also be measured. The group is currently studying alcohols (ethanol and butanol), biofuel blends, jet fuel and biodiesel surrogates. The results from these investigations will serve as validation targets for the detailed chemical kinetic models of these fuels and will provide greater insight into the fundamentals of biofuel combustion.
Left: Laser diagnostic setup for flame studies. Right: Premixed stagnation flame apparatus.
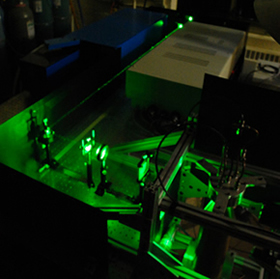 |
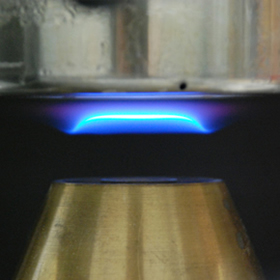 |
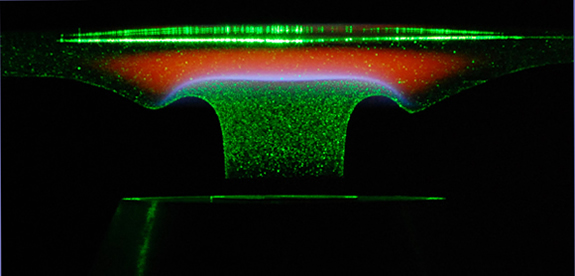
Image of stagnation flame with particles illuminated by laser sheet.
Non-premixed flame studies of alternative and traditional fuels:
Advancing the understanding of high-temperature oxidation reactions that occur in many industrial applications is a significant challenge facing combustion research. Doing so is a key step in optimizing fuel consumption and reducing pollutant formation, in combustion processes generated by fossil or alternative fuels. Studying the combustion of these fuels in an opposed flow diffusion flame allows measurements of critical properties such as extinction strain rate and species concentration profiles using laser diagnostics and chromatography techniques. This experimental data, in addition to that reported in literature, can be used to validate detailed kinetic models at high temperature and further develop these models to predict the observed experimental results. Current fuels under study include jet fuel surrogates, comprised of alkane, alkyl cyclane, and alkyl benzene compounds, and biojet surrogates, which includes methyl palmitate, methyl oleate and methyl linoleate.
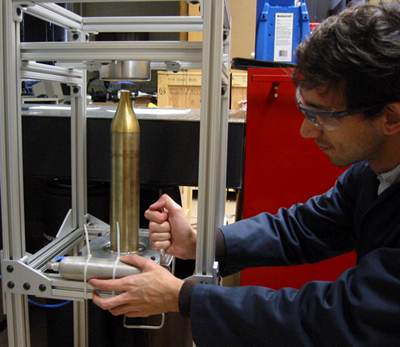
Alternative-fuel ignition:
Another important combustion property that must be understood before fuels can be efficiently used in automotive, aircraft, or power-generating engines is the auto-ignition property of the fuel, which is similar to the well known Octane and Cetane ratings of gasoline and diesel fuels. Towards this goal, the ignition properties of alcohols and biodiesel-surrogate fuels are being studied in a shock-tube facility to develop models for alternative-fuel ignition. Our research has shown that the ignition properties of a biodiesel surrogate, methyl butanoate, are similar to that of diesel fuel, n-heptane, under stoichiometric conditions and important differences under fuel rich conditions. Such studies will allow the prediction of ignition in advanced homogeneous-charge compression-ignition (HCCI), diesel, or gas-turbine engines.
Schematic of shock-tube ignition facility.
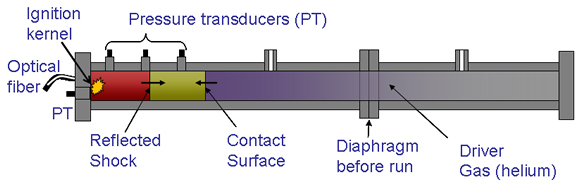
Stirling-engine burner technologies for biomass cogeneration:
Cellulosic biomass is an important energy feedstock that can reduce global emissions of CO2. Such biomass can be gasified into synthesis gas (a mixture of hydrogen and carbon monoxide) and then the synthesis gas can be further burned cleanly to cogenerate electric power and heating for residential or industrial applications. Novel burners that utilize heat recirculation by a solid such as a porous medium or through contact with the burner solid walls can be employed to generate a zone of excess enthalpy in the flame to allow the combustion of extremely lean mixtures of fuel and air that result in low soot particulate and nitrogen oxide emissions. In this type of process, phenomena such as hydrodynamic and thermodynamic effects, heat transfer between fluid and wall, radical quenching, and surface reactivity take place. This work aims at improving the understanding of the numerous phenomena involved in these excess enthalpy flames. The leading order effects influencing the flame behaviour are determined by performing experiments to validate models. Such a burner is ideally suited to coupling with a stirling engine for silent electric power generation.
Flames are studied in small channels. Left: Experimental apparatus. Right: Image of flame.
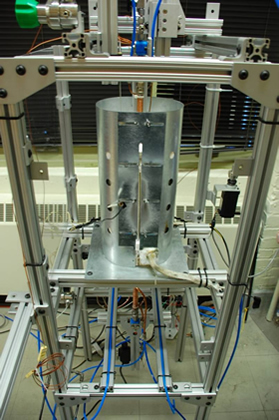 |
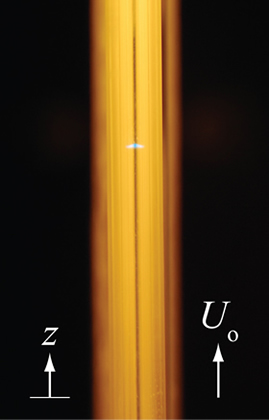 |
Metal-water reaction for hydrogen production:
Hydrogen is a promising renewable fuel source, yet it's use is limited by difficulty in transporting and storing it at a sufficiently high energy density. A new hydrogen production method involving the oxidation of reactive metal powders is being investigated. Reactive metals are capable of producing large amounts of hydrogen in a short period of time for emergency use through a reaction with water at temperatures below 70 C. This means of hydrogen production is ideal for emergency power generation. Our study focuses on temperature and particle size effects on hydrogen production rate and total yield, as well as examining reaction mechanisms effecting these results.
"Hybrid" flames of gaseous and solid fuels:
The reaction of particulate suspensions of light metals, with the products of hydrocarbon flames is one of the critical stages in the combustion of solid and metalized gelled propellants, pyrotechnics, and metalized explosives. Other hybrid mixtures are also found in process industries and in the mining industry, where dust explosions are often enhanced by the presence of hydrocarbon fuels. The majority of experimental work in this area is focused on the combustion of large (tens of microns) individual particles and agglomerates or on the combustion of specifically formulated solid propellant compositions with very low metal particle loading. It is often implicitly assumed that the results can be extrapolated to smaller particles and dense suspensions which are found in applications like explosions in dust clouds and in propellant compositions. However, these studies ignore flame front propagation in the bulk suspension and the effect of concentration on the reaction of the metal.
One of our key findings is the ability of the solid-fuel flame front to couple to the gaseous-fuel flame front with increasing solid fuel concentration. In aluminum-methane fuels, the transition to fast oxidation of aluminum is marked by the formation of an aluminum flame front which moves down and couples with the methane flame front. In iron-methane mixtures, this flame secondary metal flame front is separated by a dark zone.
Left: Aluminum-CH4-air with increasing concentration. Right: Stabilized iron-air methane flame showing two flame fronts
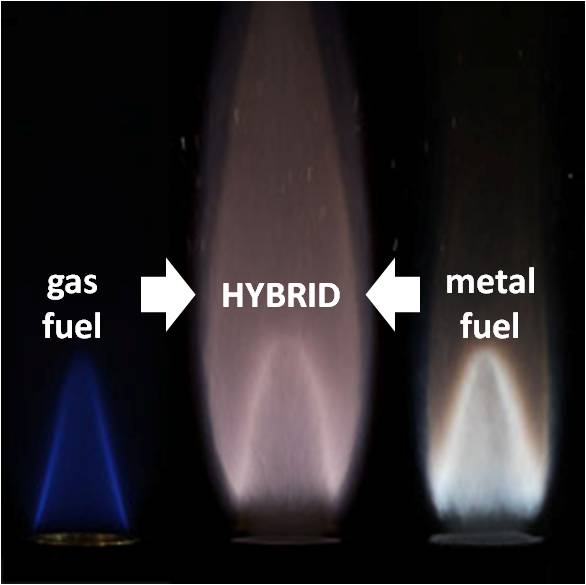 |
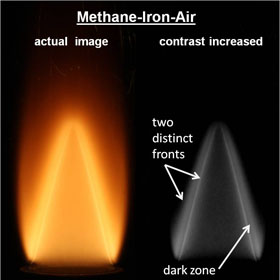 |
Transition from slow oxidation of aluminum to fast aluminum combustion manifested by aluminum flame front formation
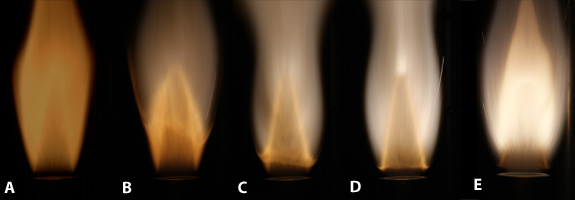
Metal Powders as Recyclable Zero-emission Energy Carriers:
Green and renewable energy sources, such as solar, wind or hydroelectric, will supply an increasing portion of the world’s electrical requirements. However, output from these sources is irregular due to daily (wind or solar) or seasonal (hydro) fluctuations and even their combined production rarely matches residential and industrial demand. Furthermore, electricity from these renewables cannot be used directly for transportation. An energy carrier is needed for storing energy for transportation and portable/remote power applications. The ideal energy carrier should be inexpensive, safe for the environment, health and routine handling, and should be widely available to meet national consumption.
This research proposes a new method for using metal powders, such as iron and aluminum, as energy carriers. These powders have energy densities equal to or exceeding hydrocarbon fuels and have an energy storage capacity that is more than a factor of ten greater than batteries. The powders are benign, abundant, and cheap. Moreover, they react with air to produce solid metal-oxide particles and they have a moderate combustion temperature compatible with external combustion engine technologies. Combustion products can be captured with existing cyclonic separation technology and recycled back to metal using clean energy, resulting in a completely closed-loop energy-carrier cycle.
The metal energy carrier economy
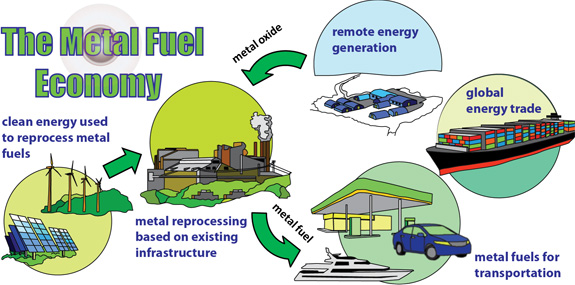
Left: energy density comparison of metals verses conventional fuel and hydrogen
Right: a turbulent iron flame and a laminar aluminum flame
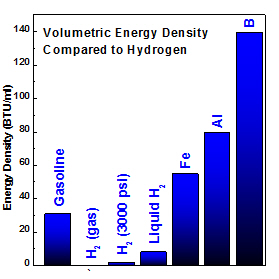 |
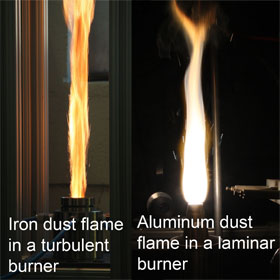 |
Vaporization of biofuel mixtures:
As biofuels are blended in increasing proportions with petroleum fuels, they will increasingly affect the formation, breakup and evaporation of the fuel spray that is the first stage of the combustion process. We are using numerical and experimental techniques to understand how mixtures of biojet and petroleum-derived jet fuel evaporate and ignite in order to assess the implications on the combustion processes in gas-turbine engines. Measurements of fuel vaporization processes for a variety of mixtures and temperatures will lead to an improved understanding of the influence of the chemical structure of the blended fuel on droplet vaporization.
|